The most effective bridge design depends on the specific situation. However, truss bridges are often considered highly efficient for their strength-to-weight ratio. Truss bridges use a series of connected triangles to distribute forces evenly, making them ideal for long spans and heavy loads. They are commonly used for railway bridges and highway overpasses.
Other effective designs include arch bridges, which excel at transferring weight to supports at each end, and suspension bridges, which can span great distances. Each type has its strengths and weaknesses, and engineers must carefully analyse the requirements of each project to determine the best solution. The design choice can significantly impact a bridge’s longevity, safety and cost.
Fundamental Principles of Bridge Design
Bridge design relies on fundamental principles that ensure structures can withstand loads and environmental forces. These principles focus on load capacity, force distribution, and proper material selection to create safe and durable bridges.
Load-Bearing Capacity
Load-bearing capacity is crucial for bridge stability. Engineers must calculate the maximum weight a bridge can support, including its weight and expected traffic loads.
Static loads come from the bridge’s structure and permanent fixtures. Meanwhile, dynamic loads result from moving vehicles, wind and earthquakes.
Safety factors are built in (to account for unexpected stresses). These factors ensure the bridge can handle loads beyond normal conditions.
Designers use computer models to simulate various load scenarios. This helps identify weak points and optimise the structure before construction begins.
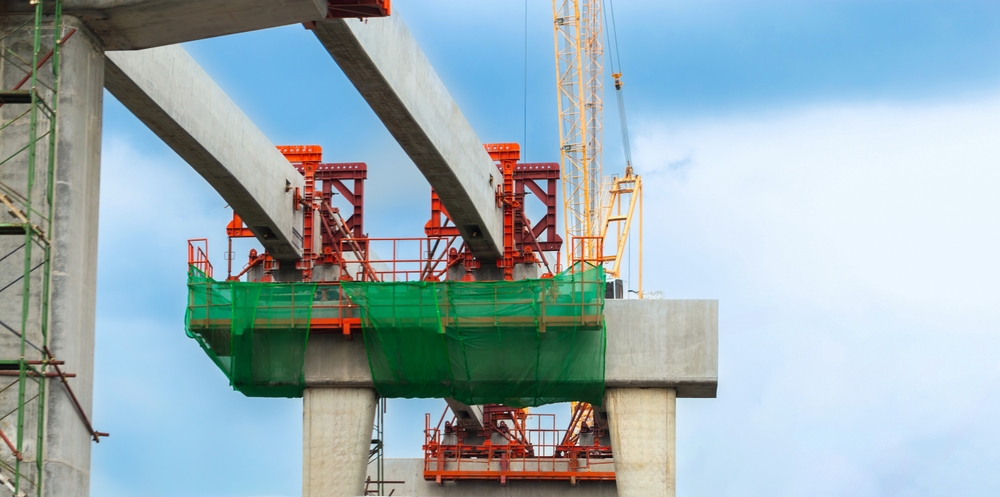
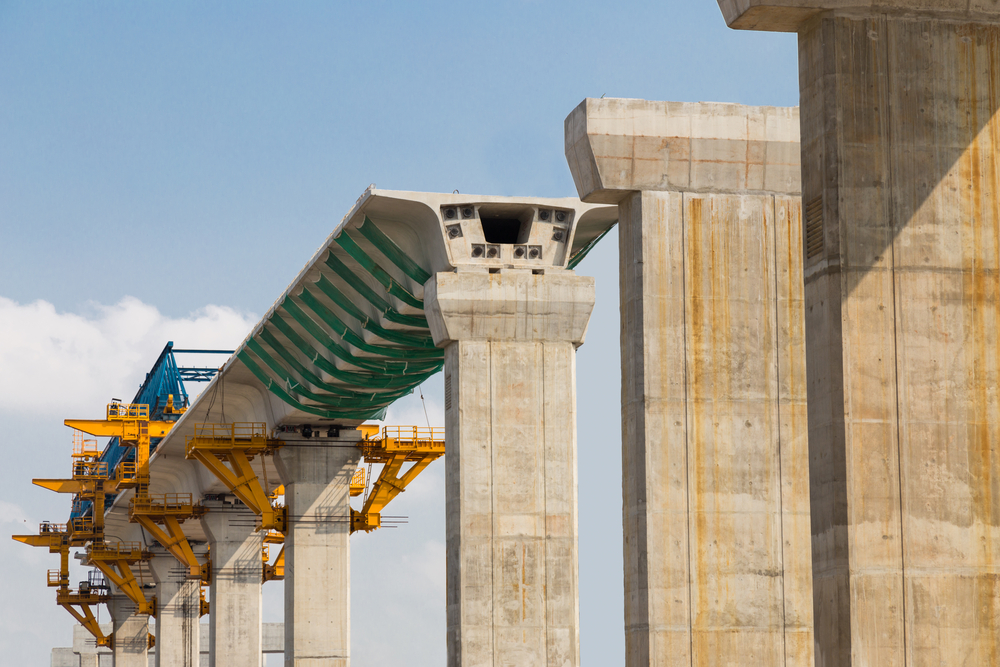
Force Distribution
Effective force distribution is vital for bridge integrity. Different bridge types handle forces uniquely.
For example, arch bridges excel at compressive force management. The arch shape transfers loads to the abutments at each end.
Meanwhile, suspension bridges use cables to distribute tensile forces. These cables transfer loads from the deck to the towers and anchorages.
Lastly, truss bridges employ a network of connected elements. This design distributes forces evenly across the structure.
Engineers must also consider both vertical and horizontal forces. For example, wind loads can cause significant lateral stress on tall structures.
Material Selection
Choosing suitable materials is essential for bridge longevity and performance. Common materials include steel, concrete and composites.
Steel offers a high strength-to-weight ratio and flexibility. It’s ideal for long-span bridges but requires regular maintenance to prevent corrosion.
Conversely, concrete provides durability and compression strength. Reinforced concrete combines these properties with steel’s tensile strength.
Meanwhile, modern composite materials offer lightweight strength. These can be used for specific bridge components or entire structures.
Material selection depends on span length, environmental conditions and budget. Engineers must balance strength, cost and maintenance requirements.
Innovations in materials science continue to expand design possibilities. New high-performance concrete and steel alloys offer improved strength and durability.
Types of Bridges
Bridges come in several designs, each suited for different spans and loads. Engineers choose the most effective type based on cost, materials and location. The seven main bridge types each have unique strengths and applications.
Beam Bridges
Beam bridges are the simplest type of bridge structure. They consist of horizontal beams supported at each end by piers. The weight of the deck and traffic pushes straight down on the beams and piers.
Beam bridges work well for short spans, typically under 80 metres. They’re cost-effective and quick to build. Motorway overpasses often use this design.
Engineers add more supports or use stronger materials for longer spans. Steel and prestressed concrete allow beam bridges to reach 300 metres, but beyond that, other bridge types become more practical.
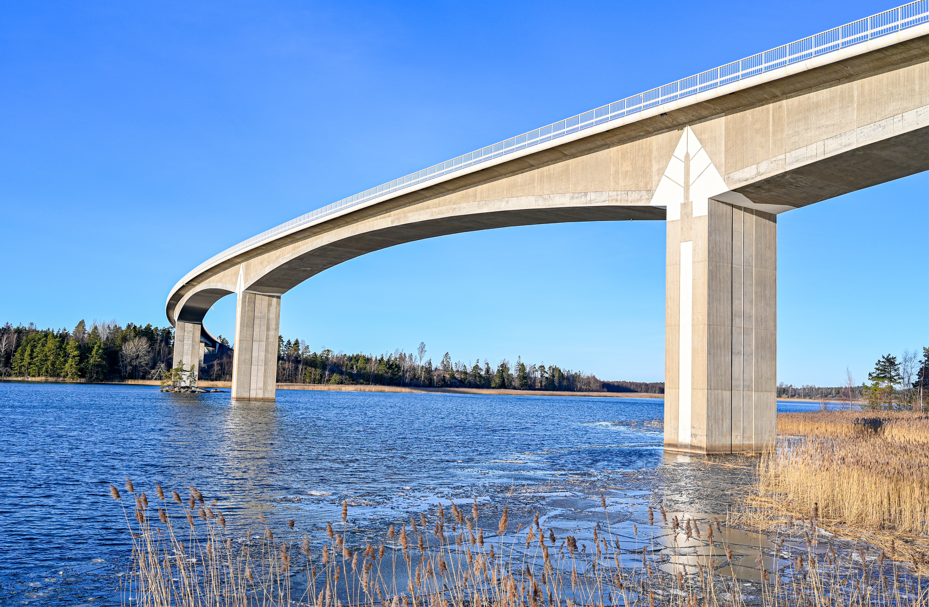
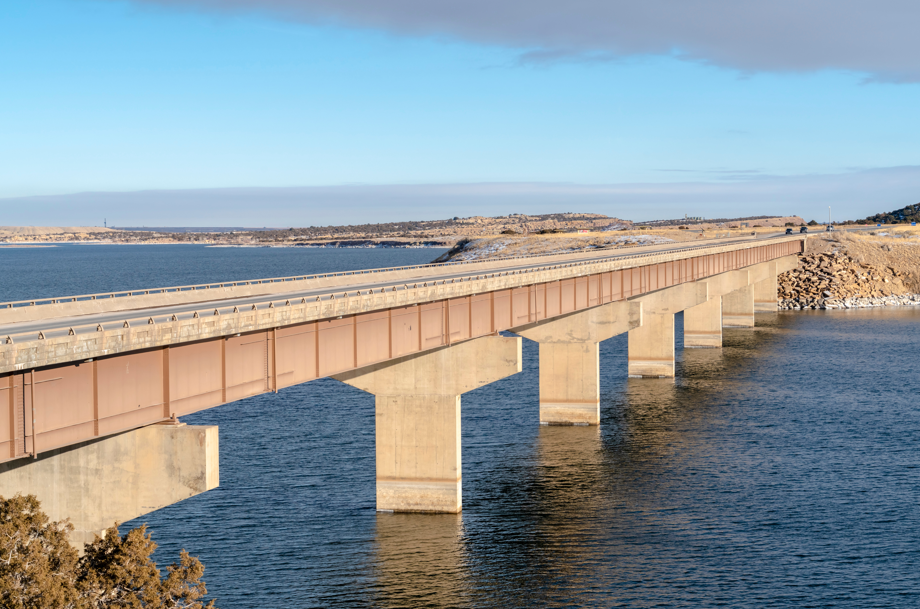
Arch Bridges
Arch bridges use curved structures to support the deck. The arch shape transfers the weight to the abutments at each end. This design is very strong and can span long distances.
Stone arch bridges have stood for centuries. Meanwhile, modern versions use steel or concrete. They can span up to 500 metres.
Arch bridges suit deep valleys or rivers with strong banks. They’re beautiful but require extensive foundations. The arch must push against solid ground to stay up.
Truss Bridges
Truss bridges use a framework of connected elements. These form triangular units that spread the load across the structure. The design is lightweight yet strong.
Engineers use trusses for medium-length spans, typically 20 to 375 metres. They’re often made of steel and can support heavy loads.
Railways frequently use truss bridges. The open design lets trains pass through the structure, keeping the deck low and stable.
Suspension Bridges
Suspension bridges can cross the longest spans. They use huge main cables anchored at each end, and smaller vertical cables hang from these to support the deck.
The main cables transfer the bridge’s weight to the towers and anchors. This design can span over 2,000 metres, and the Humber Bridge in England reaches 1,410 metres.
Suspension bridges are suitable for wide rivers or deep valleys. They use less material than other types for long spans but need massive anchorages to hold the cables.
Cantilever Bridges
Cantilever bridges use horizontal beams supported at only one end. Two cantilever arms reach out from the shores, and a central span often connects them.
This design can handle up to about 500 metres of medium to long spans. It’s useful when the middle of the gap is hard to build on.
The Forth Bridge in Scotland is a famous example. Its two 207-metre cantilever spans were a significant feat in 1890. The design is still used today for its strength and versatility.
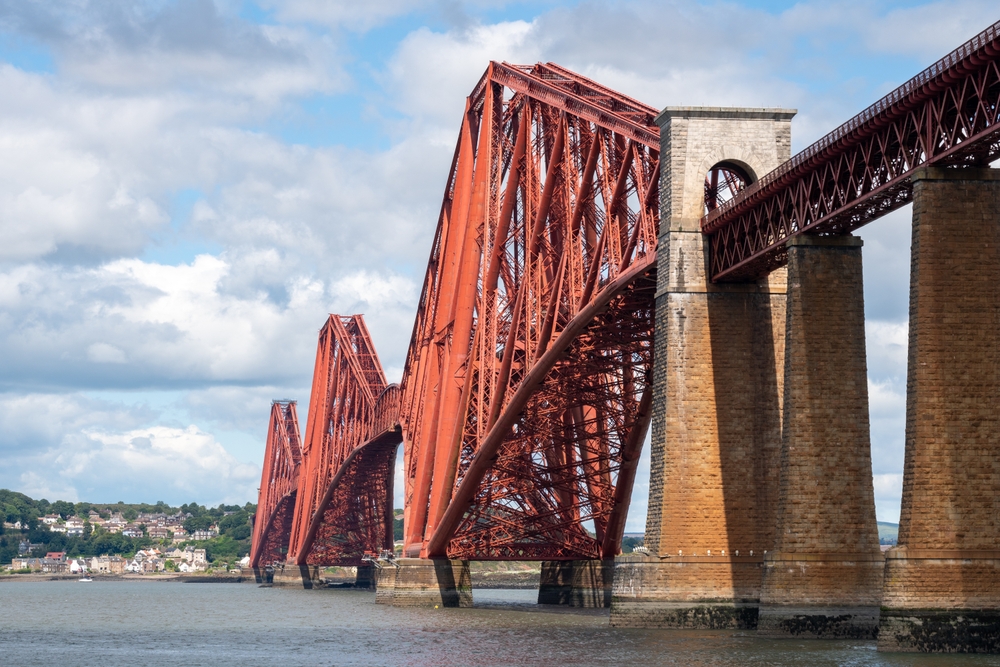
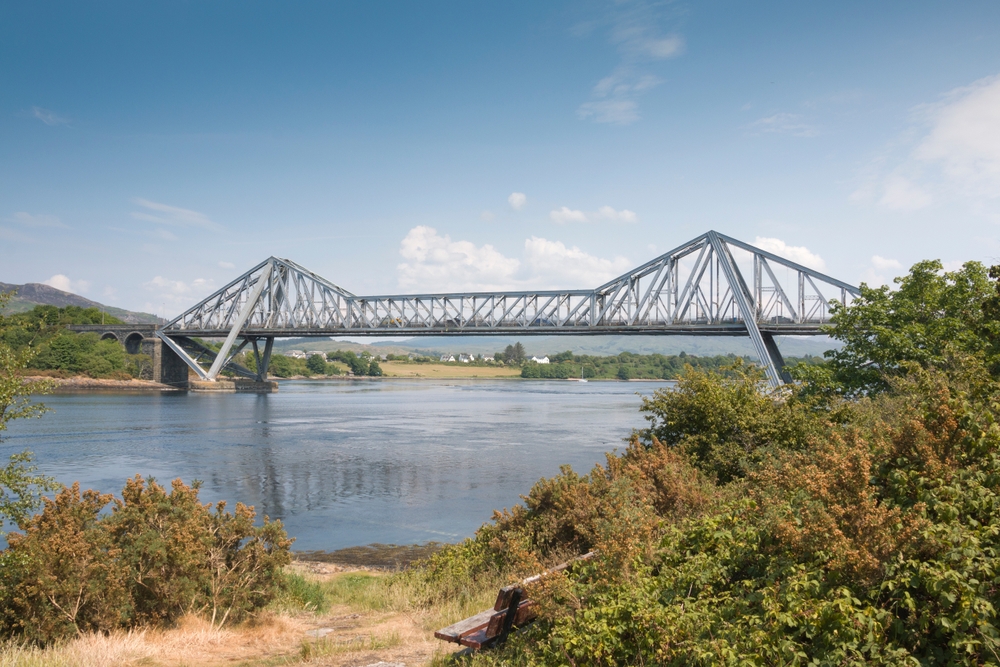
Cable-Stayed Bridges
Cable-stayed bridges use cables running directly from towers to the deck. This creates a fan-like pattern of supports.
The design is efficient and can span 500 to 1,000 metres. It uses less cable than suspension bridges and is stiffer.
Cable-stayed bridges have become popular since the 1970s. They’re attractive and work well in urban areas. The deck can be thinner than other bridge types, saving materials.
Tied-Arch Bridges
Tied-arch bridges combine arch and suspension designs. The arch rises above the deck, supported by cables or rods hanging down.
The deck acts as a tie, holding the arch ends together. This stops them from spreading out and allows the bridge to span gaps without strong side support.
Tied-arch bridges suit spans of 40 to 300 metres. They’re elegant and efficient and work well for river crossings where the banks can’t take much sideways force.
Engineering Considerations
Bridge design requires careful analysis of structural and environmental factors. Engineers must balance stability, strength and efficiency while accounting for site-specific conditions.
Stability and Strength
Successful bridge design must prioritise stability and strength. Engineers use advanced modelling techniques to analyse load distribution and stress points.
Key factors include:
- Material selection (steel, concrete, composites)
- Support structures (piers, abutments)
- Deck design and thickness
- Cable or truss configurations
Dynamic loads from traffic, wind and seismic activity must be accounted for. Engineers conduct rigorous testing to ensure the bridge can withstand expected forces over its lifespan.
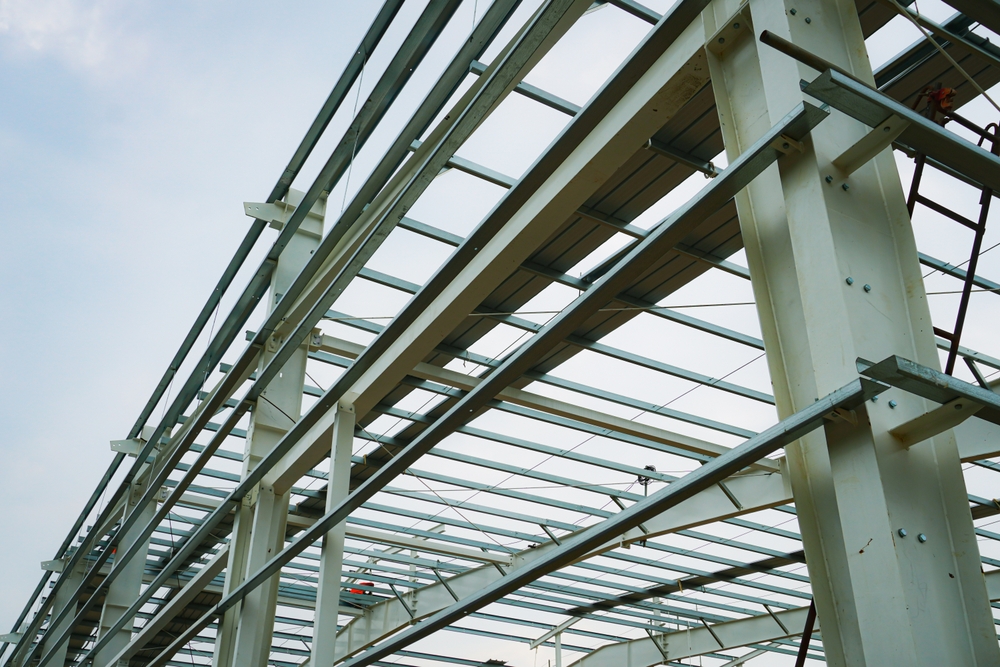
Effects of Environmental Factors
Environmental conditions significantly impact bridge performance and longevity. Engineers must consider:
- Climate (temperature fluctuations, rainfall, humidity)
- Water flow and erosion for river crossings
- Wind patterns and potential storm forces
- Seismic activity in earthquake-prone regions
Robust bridge design incorporates features to mitigate environmental risks. This may include expansion joints, corrosion-resistant materials, or specialised foundations.
Designers also assess environmental impact. They aim to minimise disruption to local ecosystems during construction and operation.
Efficiency and Construction Methods
Efficient bridge design balances performance with cost-effectiveness and constructability. Engineers consider:
- Span length and height requirements
- Available materials and transportation logistics
- Site accessibility and equipment needs
- Construction time and labour requirements
Modern construction methods, such as prefabrication and modular assembly, can reduce on-site work and improve quality control. They can also help lower costs and speed up the construction process.
Computer-aided design tools also play a crucial role. They help engineers optimise structural elements for maximum efficiency.
Engineers also evaluate long-term maintenance needs. They aim to minimise lifecycle costs through durable designs and easily replaceable components.
Frequently Asked Questions
The most effective bridge design depends on span length, load requirements and site conditions. Generally, truss and arch bridges are considered highly effective for longer spans, while beam bridges are suitable for shorter distances.
Simple beam bridges are often considered the weakest design, especially for longer spans. They lack the structural support in more complex designs, making them prone to bending and sagging under heavy loads.
Beam bridges are typically the most economical for short spans, as they require less material and are more straightforward to construct. Cable-stayed bridges, on the other hand, can be cost-effective for longer spans due to their efficient use of materials.
The strongest angle for bridge components is typically 45 degrees. This angle provides an optimal balance between vertical and horizontal forces, making it ideal for truss designs and support structures.
When selecting a bridge design, engineers consider span length, expected traffic, environmental conditions and budget factors. They use computer modelling and structural analysis to determine the most suitable option.